Two-dimensional materials are thin — only a few atomic layers thick. But their potential to change IR imaging, quantum information technology and more is huge.
HANK HOGAN, CONTRIBUTING EDITOR, HANK.HOGAN@PHOTONICS.COM
Two-dimensional materials such as graphene, as well as composite materials such as the layered semiconductor germanium selenium, could have a big impact on myriad applications.
Composite materials that act as a single-photon emitter may be valuable in quantum information technology, where being able to produce a single photon on demand enables new applications. As for graphene, it has optical properties that potentially may make it useful in commercially important areas such as the IR transceivers used for data communication.
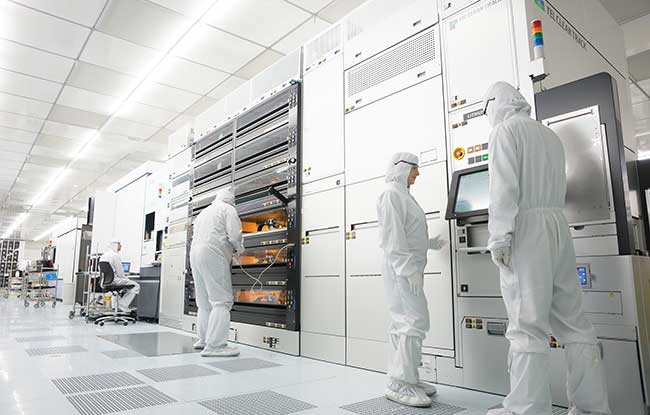
Researchers at Imec investigate the integration of graphene and other 2D materials with standard CMOS processing in the cleanroom. Courtesy of Imec.
“The fact that graphene can work at any wavelength, from the deep- and far-infrared to the visible and the UV, gives it an edge over any other material,” said Andrea Ferrari, a professor of nanotechnology at the University of Cambridge in England. He is also director of the Cambridge Graphene Centre, which is part of the Graphene Flagship; the overall goal there is to take graphene and related materials from the realm of academic laboratories into everyday life by 2023.
Other entries on the list of materials include borophene, which is made up of boron; germanene, composed of germanium; silicene, or thin layers of silicon; and composites such as tungsten disulfide or tungsten diselenide. In general, 2D materials are mechanically tough and can bend significantly without breaking. Some are good conductors or strong absorbers of light, allowing applications in flexible electronics, photonics or a combination of the two.
Graphene has attracted the most attention because of its combination of properties, some of which have led to IR transceivers with very low power consumption. Such devices typically operate at 1550 and 1300 nm. These transceivers move data through fiber optic cables over long distances between cities, as well as over the much shorter spans of a few kilometers within data centers.
While silicon photonics consumes picojoules per transmitted bit, graphene requires orders of magnitude less power — perhaps as low as 10 fJ per bit, according to Ferrari. That 1000-fold energy savings is important for data centers, which have been growing as a share of overall power consumption. That application is being targeted by the European research consortium.
“Within the Graphene Flagship, by 2020 we want to create a transceiver for 5G that works at 330 gigabits per second and, if it works, can then be incorporated in the business unit of a large telecom company,” Ferrari said.
Progress is being made toward the goal to more than triple current maximum transmission rates inside a data center, he said, adding that there are no fundamental roadblocks. However, there is a need to determine how best to incorporate graphene into standard silicon processing.

A phototransistor made up of a 2D material. There are an estimated 1000 stable 2D materials. Courtesy of Andres Castellanos-Gomez/Institute of Materials Science of Madrid.
That integration work is underway at Imec, the Leuven, Belgium-based research and development hub for nanoelectronics and digital technologies, as part of the Graphene Flagship project. Cedric Huyghebaert, R&D manager of the nanoapplications material engineering group, said that his organization is looking into how to include graphene and other 2D materials in typical CMOS manufacturing. The goal is to do so in a way that allows the process to be transferred to other groups within Imec or elsewhere. After that, the process and material would be incorporated into products.
2D challenges
Two-dimensional materials present some unique challenges. Among them: They are all surface, which makes control of the surface interface important. That is different than the silicon onto which 2D materials might be placed. Silicon is a bulk material, and therefore has only one interface. What’s more, 50 years of process engineering has resulted in good control of device properties.
Transferring a 2D material to silicon is another hurdle. Even when that is done, the thin layer of material can have trouble adhering to substrates because this is accomplished via a van der Waals interaction; this relatively weak bonding can lead to other issues as processing continues.
“When you put other layers of materials on top, [2D material] is the weakest link,” Huyghebaert said. “And it makes it very difficult to withstand temperature budgets when there is some stress buildup because you will have some delamination issues.”
Another challenge is the inability to grow large, defect-free 2D films. Silicon, again, is different, thanks to decades of R&D. Still, Huyghebaert thinks such problems will be solved. For instance, it may be possible to use circuitry to correct for optical property differences so that pixels all exhibit the same responses.
Once the fundamentals of making reliable devices are mastered, tools and techniques can be deployed that use such knowledge. Graphene and other 2D materials could then be used in hyperspectral cameras that capture images from the UV to the IR, as well as in other areas.
“I’m pretty sure [2D materials] will pop up in a lot of applications in the future,” Huyghebaert said.
One such application may lay in the far-IR, with the recent announcement of research on a graphene-based terahertz saturable absorber with an order of magnitude higher absorption modulation than other devices have previously produced.
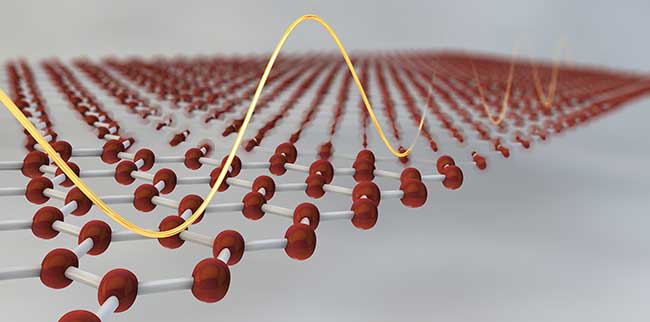
Printable graphene inks enable ultrafast terahertz lasers. Courtesy of Graphene Flagship.
A report on the work, done as part of the Graphene Flagship, appeared in the June 2017 Nature Communications paper “Terahertz saturable absorbers from liquid phase exfoliation of graphite.” According to co-author Miriam Serena Vitiello, the goal of the research is to extend the capabilities of lasers.
“We would like to integrate the developed graphene inks into the cavities of state-of-the art terahertz laser resonators, to ‘drive’ them in the ultrashort pulse regime,” she said.
Vitiello is director of research at Italy’s National Research Council and a contract professor of condensed matter physics at Scuola Normale Superiore in Pisa, Italy.
Such lasers could be used in medical diagnostics to enable detecting a tumor inside tissue. This would be done through time-of-flight imaging and take advantage of the penetration depth of terahertz waves. Another use would lie in security applications, exploiting the ability of terahertz signals to penetrate materials and thereby reveal what is hidden.
Yet another use of 2D IR materials lies in quantum information technology, thanks to the discovery that it is possible to fabricate single-photon emitters within the film. What’s more, those emitters can be precisely positioned, said Rudolf Bratschitsch, a physics professor at the University of Münster in Germany. There have been other single-photon sources, such as quantum dots or color centers in diamond, for years. Such sources are desirable in quantum information technology and elsewhere, if they are reliable, robust and produce photons on demand. Research has shown that 2D materials offer some important advantages.
2D advantages
“What is very different from all the other single-photon sources is that we can position them with strain,” Bratschitsch said. He was co-author of a related 2016 paper published in Advanced Materials — “Nanoscale Positioning of Single-Photon Emitters in Atomically Thin WSe2.” The strain arises when the 2D material is draped across nanostructures. The material conforms to the microscopic hills and valleys, creating a strain potential that serves to position the single-photon emitter at known spots. This could be next to a waveguide to get single photons, when they are produced, to where they can be used.
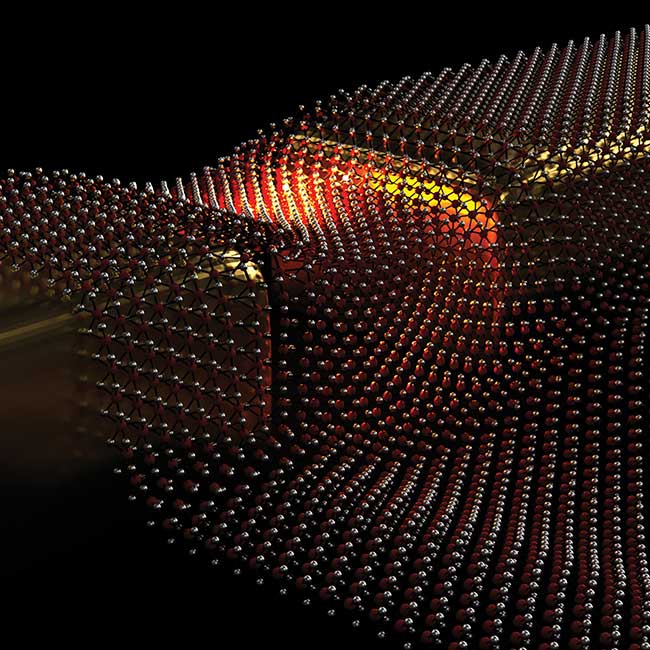
A WSe2 monolayer suspended between two gold nanorods with strain-induced light emitter in the gap. Two-dimensional materials could prove to be valuable single-photon sources. Courtesy of Robert Schmidt/University of Münster.
Bratschitsch and his group are working on a number of different materials, such as tungsten diselenide, gallium selenide and hexagonal boron nitride. At present, this is all fundamental research, as investigators try to understand the emission mechanism, wavelengths and other properties. Such research illustrates a point: There are an estimated 1000 stable 2D materials, according to Andres Castellanos-Gomez, a 2D materials and devices scientist at the Institute of Materials Science of Madrid who has collaborated with Bratschitsch.
“We just started opening the door to all the 2D materials out there,” he said. Castellanos-Gomez and his group are researching several of these materials, including black phosphorous. Unlike graphene, for which commercial-scale production exists, some of these 2D materials must be fabricated by peeling off a few layers and then making devices out of the flakes. For others, including tungsten disulfide, techniques that can grow films over large areas are already known.
With this plethora of materials, graphene may be the first used in commercial applications, but neither it nor any other 2D materials can be the sole focus of R&D efforts. Castellanos-Gomez said that so far, only a tiny fraction — some 20 out of the 1000 — of the estimated universe of stable 2D materials have been investigated. This explains the ongoing basic 2D material research happening across Europe.
“We need to have a catalog as soon as possible,” Castellanos-Gomez said, “in order to make a decision [about] where to invest our efforts.”
No comments:
Post a Comment