Schematic of the experimental setup. Infrared light (red) was focused by an atomic-force microscope tip (metallic gray) to probe the local surface chemistry on coated platinum particles (yellow) measuring about 100 nm in length. (Credit: Hebrew University of Jerusalem)
A new synchrotron infrared nanospectroscopy (SINS) study has confirmed that structural defects and jagged surfaces at the edges of platinum and gold nanoparticles are key hot spots for chemical reactivity. The experiments, conducted by a team of researchers from the ALS, Berkeley, and the Hebrew University of Jerusalem in Israel, pinpoint the most active areas of reactions on nanoscale particles and confirm that structural defects at the periphery are key to catalyst function. Experiments like this should help researchers customize the structural properties of catalysts to make them more effective in fostering chemical reactions.
Scientists have known that materials can behave differently at the nanoscale than they do at larger scales, and customizing a nanoparticle’s size and shape can enhance its properties for specific uses. The idea is that smaller particles have higher surface areas than larger particles, which means that more atoms will be located at edges. Atoms at edges have fewer neighbors than those embedded within smooth surfaces, and fewer neighbors means more freedom to participate in chemistry with other elements. However, despite the growing availability of tools that enable detailed characterization, so far it has not been possible to document this phenomenon directly. Surface properties can be mapped with high spatial resolution, and catalytic conversion can be tracked with a clear chemical signature; however, the combination of the two has rarely been achieved.
Now, with the unique SINS capability that has been developed at ALS Beamline 5.4, researchers have combined a broad spectrum of infrared light with an atomic-force microscope to reveal different levels of chemical reactivity on single nanoparticles of platinum and gold. The microscope’s tip, when coupled with highly focused infrared light, works like an extremely sensitive antenna to map the chemical properties of molecules on the surface of 100-nm-sized particles with a resolution of 25 nm.
Because chemical reactions on reactive surfaces, such as platinum nanoparticles, occur very rapidly—in less than a second—it is necessary to find an approach that will stabilize the reactive molecules on the surface, making it feasible to detect their chemical transformation. In order to achieve this goal, the researchers used a layer of chemically active molecules, which was attached to the surface of the particle, as markers for the catalytic reactivity. SINS scans across the edge of particles were compared to scans across the center. The results showed that chemical reactivity is enhanced at the edges of the particles, validating a well-known hypothesis in the field of catalysis. Also, areas with many defects at the atomic level (a function of smaller particle size) were more active than smooth surfaces.
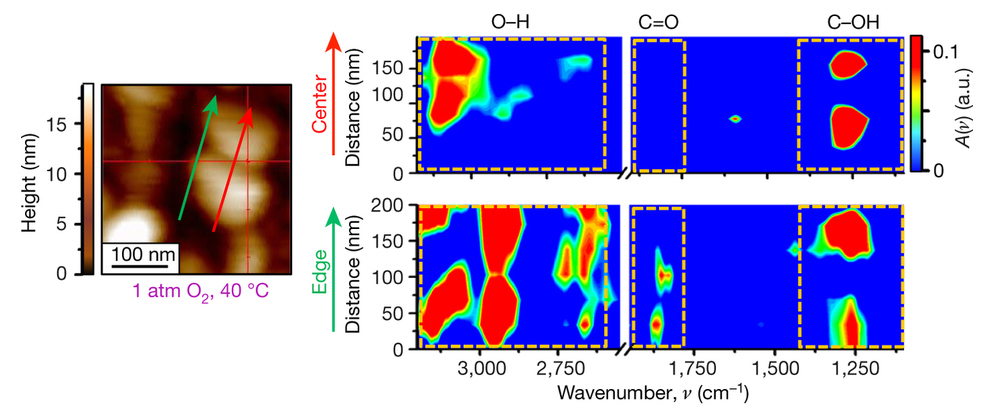
In addition to revealing where and when a chemical reaction occurs on the surface of a particle, the approach that was developed in this work will provide the tools to determine the precise level of energy that is required to trigger chemical reactions (the activation energy) at different sites. Knowing the activation energy is a key element for understanding and optimizing reactions and can reduce costs at the industrial scale by conserving energy use.
SINS is a versatile tool that can simultaneously measure reactants and products and the interplay between them with a resolution three orders of magnitude better than conventional infrared techniques. As such, it has significantly opened up the study of catalysis and surface chemistry. Future SINS experiments will focus on documenting active chemical processes by using controlled gas or liquid flows to trigger reactions; another possibility is the exploration of the effects of varying pressure and temperature. Other studies could conceivably combine infrared and x-ray methods to gather richer chemical information. There are already plans for a new infrared beamline that will increase the capacity for infrared chemical studies at the ALS and also launch infrared-based 3D structural studies.
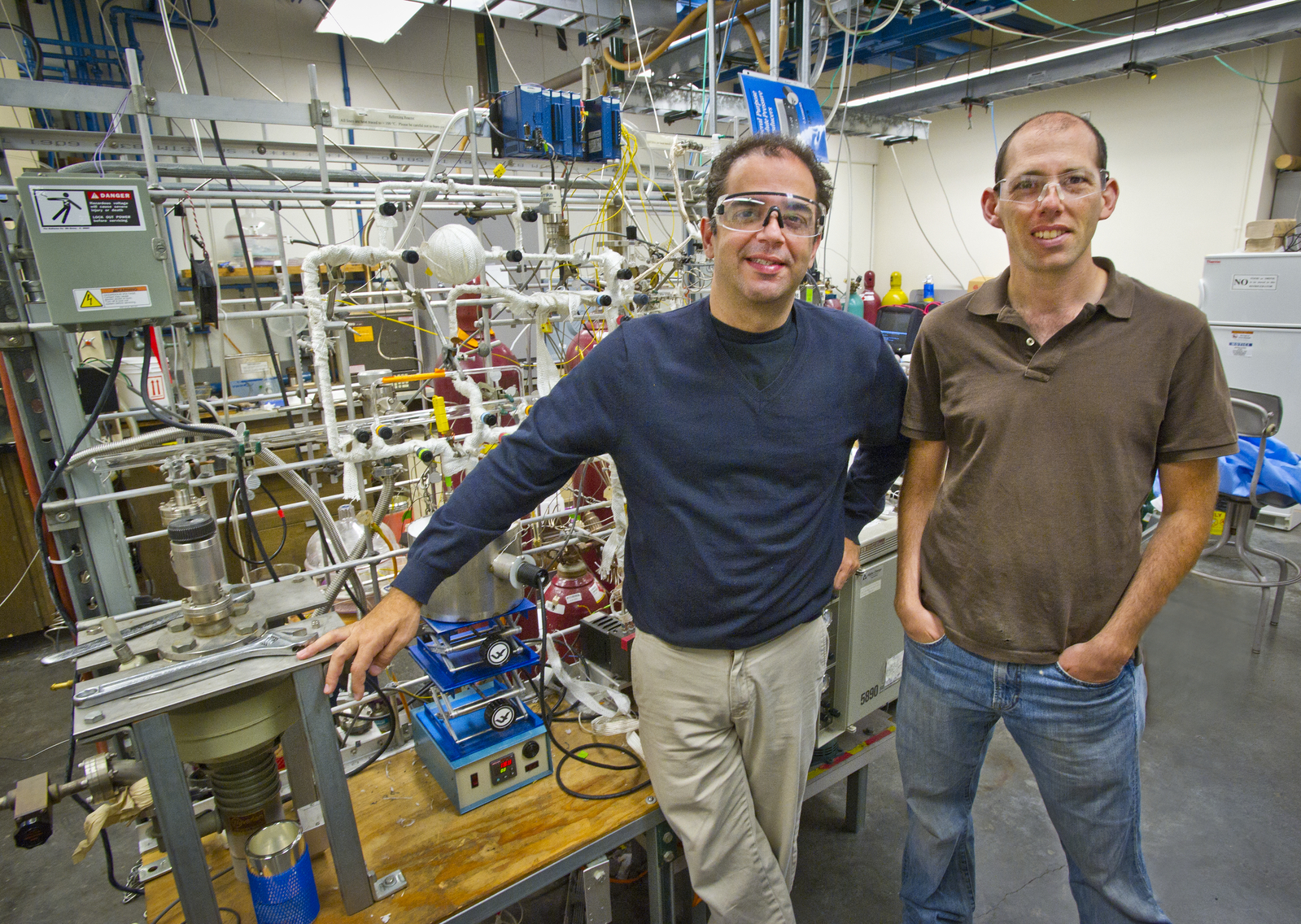
Research conducted by: C.-Y. Wu, W.J. Wolf, and F.D. Toste (UC Berkeley and Berkeley Lab); Y. Levartovsky and E. Gross (The Hebrew University of Jerusalem); and H.A. Bechtel and M.C. Martin (ALS).
Research funding: U.S. Department of Energy, Office of Basic Energy Sciences (DOE BES) and National Science Foundation. Operation of the ALS is supported by DOE BES.
Publication about this research: C.-Y. Wu, W.J. Wolf, Y. Levartovsky, H.A. Bechtel, M.C. Martin, F.D. Toste, and E. Gross, “High-spatial-resolution mapping of catalytic reactions on single particles,” Nature 541, 511 (2017). doi:10.1038/nature20795
No comments:
Post a Comment